Introduction
The constant turn rate and constant velocity model (in short CTRV) is used to model vehicles. Using the function $x_{k \vert k} = f(x_k, \nu_k)$ the model predicts the new state $x_{k \vert k}$ of the vehicle at time $k+1$ from the state $x_{k}$ and the noise vector $\nu_k$ at the current time step $k$. In addition to a constant velocity the model assumes also a constant turn rate which makes it more accurate than the constant velocity model, especially in curves.
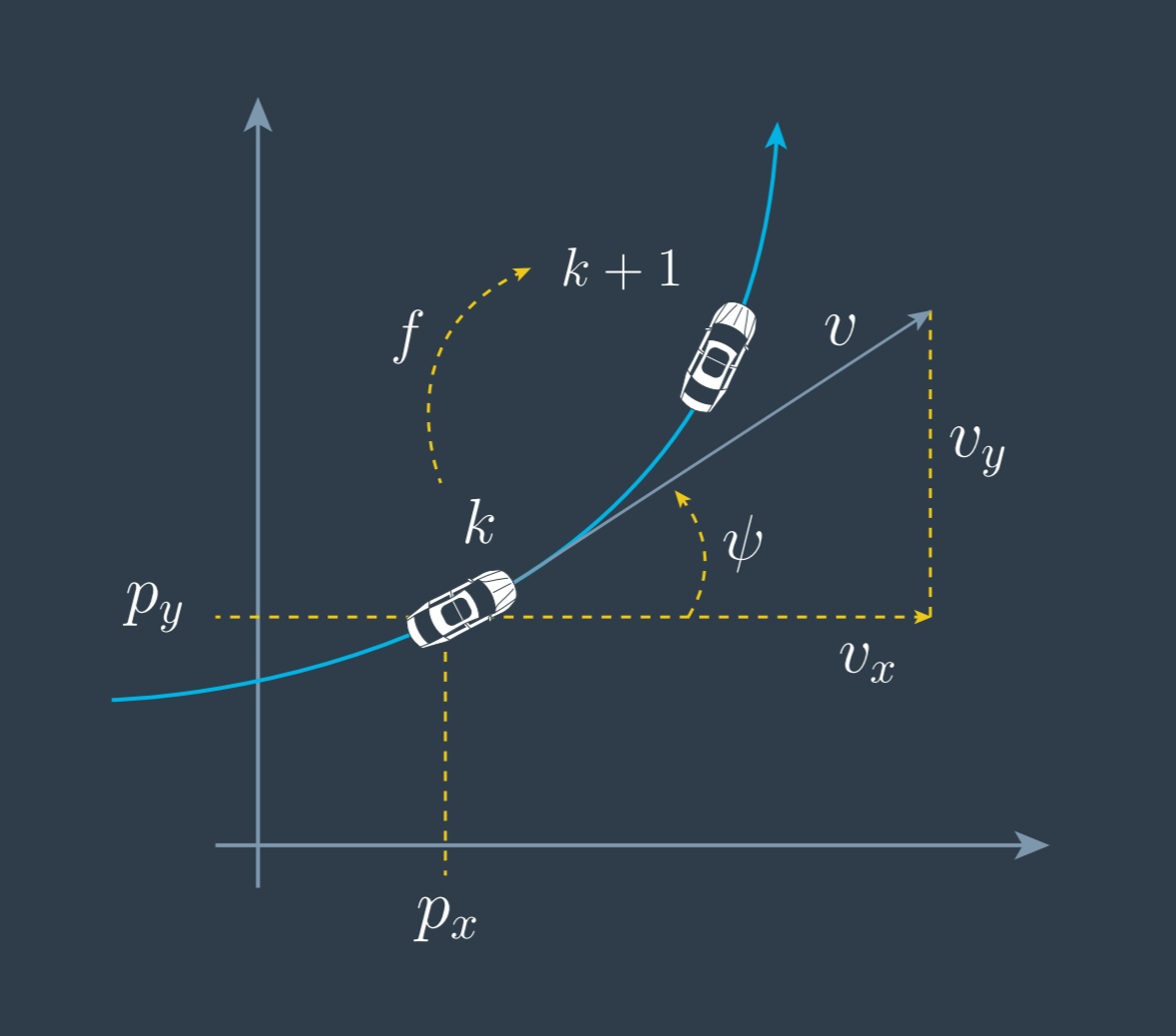
State Vector and Process Model
The state vector of the ctrv model is given as
\[x = \begin{bmatrix} p_x & p_y & v & \psi & \dot \psi \end{bmatrix}^T\]To derive the process model we investigate in the change rate of the state $x$, which is called $\dot{x}$. From the geometric relations, shown in the image above, we find how the change rate $\dot{x}$ depends on the state $x$, which is a differential equation $\dot{x} = g(x)$. To derive this differential equation the goal is to express the five time derivatives of the state, in dependency of any of the state elements.
\[\dot{x} = \begin{bmatrix} \dot{p}_x \\ \dot{p}_y \\ \dot{v} \\ \dot{\psi} \\ \ddot{\psi} \end{bmatrix} = \begin{bmatrix} v \cdot \cos{\psi} \\ v \cdot \cos{\psi} \\ 0 \\ \dot{\psi} \\ 0 \end{bmatrix}\]Obviously the change in velocity and turn rate is zero because this is the underlying assumption of the ctrv model. A constant velocity $v$ and a constant turn rate $\dot{\psi}$ is not changing. Put in mathematical terms, the derivative of a constants is zero.
Discrete State Prediction
The discrete time step $k$ relates to the continuous time value $t_k$. To get from the discrete time step $k := t_k$ to $k+1 := t_{k+1}$ we make use of the time difference $\Delta t = t_{k+1} - t_{k}$ and integrate the change rate $\dot{x}$ of the state $x$ over this time period. The result of this integral is added to the current state $x_k$.
\[x_{k+1} = x_k + \int_{t_k}^{t_{k+1}} \begin{bmatrix} \dot{p}_x \\ \dot{p}_y \\ \dot{v} \\ \dot{\psi} \\ \ddot{\psi} \end{bmatrix} \mathrm{d} t\]To solve this integral, every row of the change rate vector can be integrated.
\[\begin{align} \int_{t_k}^{t_{k+1}} \begin{bmatrix} \dot{p}_x \\ \dot{p}_y \\ \dot{v} \\ \dot{\psi} \\ \ddot{\psi} \end{bmatrix} \mathrm{d} t &= \begin{bmatrix} \int_{t_k}^{t_{k+1}} \dot{p}_x \mathrm{d} t \\ \int_{t_k}^{t_{k+1}} \dot{p}_y \mathrm{d} t \\ \int_{t_k}^{t_{k+1}} \dot{v} \mathrm{d} t \\ \int_{t_k}^{t_{k+1}} \dot{\psi} \mathrm{d} t \\ \int_{t_k}^{t_{k+1}} \ddot{\psi} \mathrm{d} t \\ \end{bmatrix} = \begin{bmatrix} \int_{t_k}^{t_{k+1}} v \cdot \cos{\psi} \mathrm{d} t \\ \int_{t_k}^{t_{k+1}} v \cdot \sin{\psi} \mathrm{d} t \\ \int_{t_k}^{t_{k+1}} \dot{v} \mathrm{d} t \\ \int_{t_k}^{t_{k+1}} \dot{\psi} \mathrm{d} t \\ \int_{t_k}^{t_{k+1}} \ddot{\psi} \mathrm{d} t \\ \end{bmatrix} \\ &= \begin{bmatrix} \int_{t_k}^{t_{k+1}} v \cdot \cos{\psi} \mathrm{d} t \\ \int_{t_k}^{t_{k+1}} v \cdot \sin{\psi} \mathrm{d} t \\ \int_{t_k}^{t_{k+1}} 0 \mathrm{d} t \\ \int_{t_k}^{t_{k+1}} \dot{\psi} \mathrm{d} t \\ \int_{t_k}^{t_{k+1}} 0 \mathrm{d} t \\ \end{bmatrix} = \begin{bmatrix} \int_{t_k}^{t_{k+1}} v \cdot \cos{\psi} \mathrm{d} t \\ \int_{t_k}^{t_{k+1}} v \cdot \sin{\psi} \mathrm{d} t \\ 0 \\ \int_{t_k}^{t_{k+1}} \dot{\psi} \mathrm{d} t \\ 0 \\ \end{bmatrix} \end{align}\]
Comments